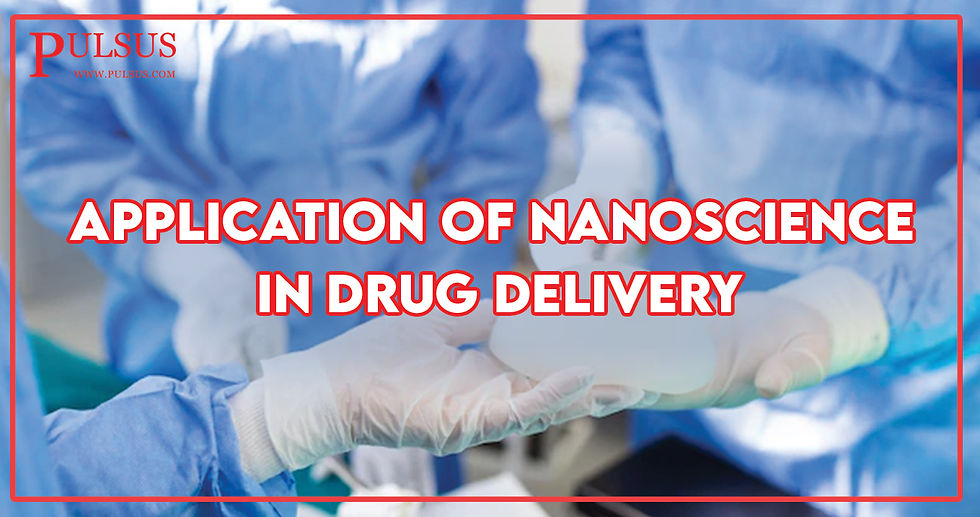
Numerous interesting opportunities in healthcare are being presented by the use of nanotechnology in medicine, or nanomedicine. In this article, we focus on two crucial facets of nanomedicine: drug delivery and tissue engineering. We emphasize the recent developments, the difficulties we are now facing, and what is likely to happen in the near future.
A novel molecular entity (NME) that has strong biological activity but poor water solubility or a very short half-life will probably face substantial development hurdles or be declared undevelopable in the pharmaceutical sectors. On the other hand, pharmaceutically superior substances that are less active might prove to be better prospects for development. There is always some degree of compromise in both situations, and these trade-offs may unavoidably lead to the creation of less-than-ideal medications. However, some of the drawbacks related to potential NMEs can now be addressed because of recent breakthroughs in nanotechnology and future trends. The pharmacological properties (such as solubility and circulation half-life) of such NMEs can be significantly enhanced by using nanoscale delivery vehicles, essentially leading to the development of the most ideal safe and effective medication candidates. This is only one illustration of how nanotechnology may fundamentally alter the procedures and opportunities for drug discovery as well as the structure of the pharmaceutical businesses. In fact, the advancement of already-approved medications has verified the efficacy of current nanotechnology-based therapeutic solutions, and other innovative classes of nanotherapeutics are currently being developed.
In addition to enabling new therapeutic classes and encouraging the re-investigation of previously unfeasible new molecular entities that are pharmaceutically suboptimal but biologically active, nanoscale delivery vehicles can improve the therapeutic efficacy and minimize side effects associated with existing medications.
Nanoscience-based sensors, such as those made of nanowires, nanotubes, nanoparticles, cantilevers, and micro-/nanoarrays, can quickly and efficiently identify disease biomarkers while requiring fewer samples. Additionally, single-cell analysis and the early detection of bacteria, viruses, and circulating tumor cells are both made possible by nanoscience.
Certain nanomaterials can treat diseases directly because they possess special therapeutic qualities that set them apart from traditional medicines. For instance, gold nanoshells/nanorods, carbon nanotubes, and magnetic nanoparticles can cause hypothermia to kill cancer cells, and nanocrystalline silver is being utilized as an antibacterial agent. Hafnium oxide and gold-based nanoparticles can also significantly improve X-ray therapy.
Nanoscience and Nanomedicine have had a tremendous impact on the creation of drug delivery systems ever since liposomes were proposed as carriers of proteins and medications for the treatment of disease. In order to create efficient therapeutic modalities, a number of organic and inorganic nanomaterials and devices have been used as delivery systems. Others than two dozen nanotechnology-based medicinal items have so far received FDA approval for use in clinical settings, and more are now undergoing clinical studies. These goods are classified as first-generation nanotherapeutics because the bulk of them consist of medicine plus an untargeted delivery vehicle (such as polymers and liposomes).
The first generation of nanoscience offers several benefits over traditional drug delivery. For example, they can improve therapeutic action by extending drug half-life, enhancing hydrophobic drug solubility, lowering possible immunogenicity, and/or releasing pharmaceuticals either continuously or in response to stimuli. As a result, both the frequency of administration and the hazardous side effects of medications can be decreased. In addition, due to the increased permeability and retention effect, nanoscale particles might passively gather in a particular tissue. Beyond these clinically effective nanosystems, next-generation nanoscience for "smart" medication delivery has been developed using nanotechnology to support new therapeutics.
The two most recent and well-known medicines, gene therapy and RNA interference (RNAi), show significant potential for both treating and preventing a variety of disorders. However, a number of hurdles, including enzymatic degradation, reticuloendothelial system absorption, renal filtration, and restricted intracellular penetration, impact the systemic administration of novel therapies. Therefore, it has become common practice to encapsulate and enhance the effectiveness of these novel treatments using cationic nonviral nanocarriers. Unfortunately, a large number of these substances have the potential to cause harmful side effects such as toxicity, immunological or inflammatory responses, and serum instability, which renders them unfit for use in clinical settings. Consequently, the barrier to the widespread application of RNAi and gene therapy is the lack of safe and efficient delivery materials.
Combination therapy has demonstrated a number of possible benefits and could be more successful than single-medication therapy. However, the synergistic drug ratio optimized in vitro will surely change following the traditional administration of medicine, which could then result in insufficient therapeutic outcomes in vivo. This is because different pharmaceuticals have different pharmacokinetic characteristics. To this goal, co-delivery has been made easier by the application of lipid- and/or polymer-based nanoscale systems, which were previously designed for the delivery of a single medication. When using certain drug combinations, we are able to transport the pharmaceuticals to the target areas concurrently while successfully adjusting the relative dosage of the different treatments at the level of the single particle.
The goal of the rapidly developing interdisciplinary subject of tissue engineering, which combines biology, engineering, materials science, and medicine, is to create biological substitutes that can preserve, improve, or replace the function of tissues and organs. The development of implantable tissues during the past few decades, some of which are now being tested on humans, is the result of continuous advancement in this particular sector. However, the majority of tissue engineering techniques are based on the idea that, under the right bioreactor settings, cells that have been seeded or recruited into three-dimensional (3D) biocompatible scaffolds can reassemble into useful structures that resemble genuine tissues. Early man-made scaffolds attempted to provide cells with structural integrity on a macroscopic level, but they were only somewhat successful.
Comments